Which microscopes in the BIDC are capable of 2-photon imaging?
Nikon A1R:
The Nikon A1R is an upright 2-photon laser scanning microscope. Designed for intravital and explant deep tissue imaging, this microscope includes a perfusion system with an in-line stage heater and an anesthesia setup. The Nikon A1R utilizes one MaiTai Deep See IR laser and an ultra-sensitive gallium arsenide phosphide non-descanned detector (GaAsP NDD) to take sharp images deep within a sample. The 2-photon microscope is built to accurately image in three channels (Hoechst, FITC, TRITC). Additionally, the Nikon A1R features a 1K resonant scanner capable of imaging at either an ultrahigh-resolution of up to 4096 x 4096 pixels (15 frames per second) or at an ultrahigh-speed of up to 420 frames per second (512 x 32 pixels). This hybrid scanner makes it possible to use one microscope in order to visualize clear images with fine details as well as capture quick cell interactions and movements deep within a tissue. Plus, the ultrahigh-speed scanner images with a short dwell time which limits photobleaching, phototoxicity, and sample damage.
This instrument uses the NIS-Elements
software. More information can be found here.
Analyzing images from the Nikon A1R can be performed by using the image analysis software Imaris
. NIS-Elements exports images as .nds files, which can be quickly converted to Imaris files using the Imaris File Converter
. Images and movies are then easily imported into Imaris and ready for further processing. The BIDC holds two Imaris licenses that are available on our Analysis Stations. Anyone can use our stations by signing up and booking time on the equipment. A more detailed work through of image analysis can be found here.
A1R Filter Set:
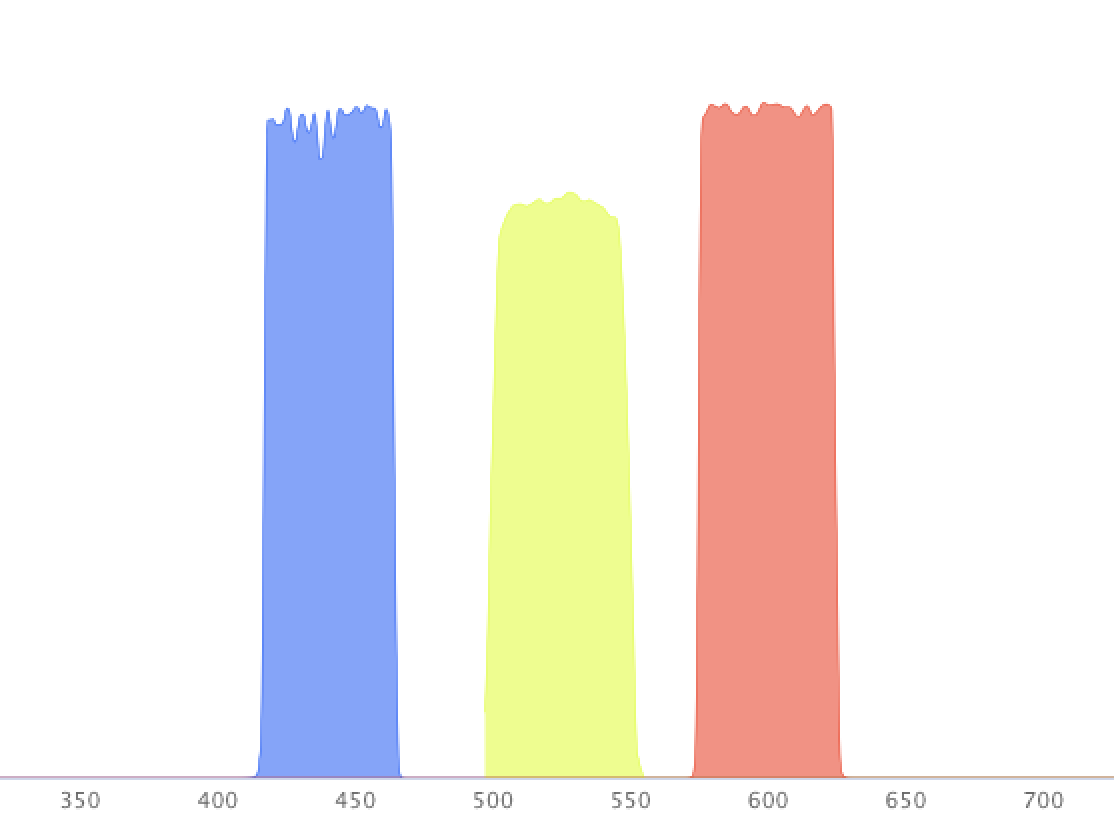
Generation 3 (“Gen 3”):
The "Gen 3" is the third generation of custom upright 2-photon laser scanning microscopes designed and built by the BIDC. Constructed for intravital and explant deep tissue imaging, this microscope includes a perfusion system with an in-line stage heater and an anesthesia setup. The “Gen 3” claims two IR lasers: an 18W Coherent Chameleon Vision II (680nm - 1080nm) and an 8W MaiTai Ti-Saphire (710nm - 920nm). The instrument features an impressive array of 6 filter sets and 6 H9433MOD photomultiplier tubes (PMTs), which have a high signal to noise ratio. This set up allows for the simultaneous detection of violet, blue, green, yellow, red, and far red dyes at video rate. The 6 filter sets are as follows: dichroic 458 (transmitted 417/60), dichroic 490 (transmitted 475/23), dichroic 520 (transmitted 510/42), dichroic 568 (transmitted 542/27), dichroic 650 (transmitted 607/70), and dichroic 725 (transmitted 675/67). The custom microscope makes it possible to design and image a remarkably large and reliable panel in live tissues.
This instrument is controlled by a specialized Micro-Manager
plugin called µMagellan. More information about µMagellan can be found in the article here, and the µMagellan user guide can be found here.
Similar to the Nikon A1R, analyzing images from “Gen 3” can also be performed by using Imaris
. However, µMagellan exports data as a TIF and must be converted to an Imaris file by using a FIJI
plugin called Imaricumpiler. Then, the data can be imported into Imaris and analyzed. For more details, please refer to our Analysis Stations and Image Analysis pages.
Gen 3 Filter Set:
Gen 3 Microscope Layout:
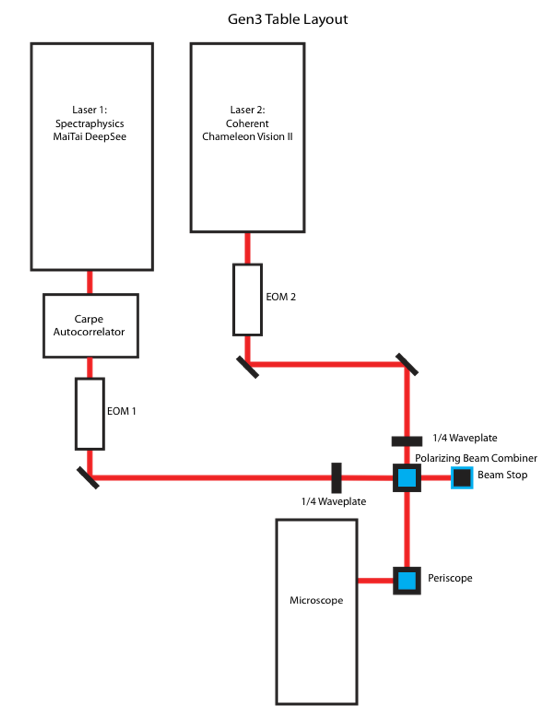